A Shot Under Pressure
Activity courtesy of TeachEngineering.org, a teacher-tested, searchable online library of standards-based lessons and activities developed by the Integrated Teaching and Learning Program at the University of Colorado’s College of Engineering. See companion activity on Bernoulli’s Principle.
Note: Teachers should err on the side of caution and make sure administrators know about and approve of letting students bring their water guns to school.
Summary
Working in groups of three, high school students use their understanding of projectile physics and fluid dynamics to calculate the water pressure in squirt guns by measuring the range of the water jets. They create graphs to analyze how the predicted pressure relates to the number of times they pump the water gun before shooting.
Grade level: 9-12
Time: 120 minutes
Cost: $2 per group
Learning Objectives
- Use projectile motion physics to determine the initial velocity of a projectile launched horizontally.
- Use Bernoulli’s equation to find the pressure of a fluid.
- Collect, record, and analyze data to determine relationships among variables.
Learning Standards
Common Core State Math Standards
- Create equations that describe numbers or relationships. Rearrange formulas to highlight a quantity of interest, using the same reasoning as in solving equations. For example, rearrange Ohm’s law V = IR to highlight resistance R.
- Solve linear equations and inequalities in one variable, including equations with coefficients represented by letters.
- Represent and solve equations and inequalities graphically. Understand that the graph of an equation in two variables is the set of all its solutions plotted in the coordinate plane, often forming a curve (which could be a line).
- Solve equations and inequalities in one variable.
Next Generation Science Standards
Create a computational model to calculate the change in the energy of one component in a system when the change in energy of the other component(s) and energy flows in and out of the system are known. [9 – 12]
International Technology and Engineering Educators Association
Energy cannot be created nor destroyed; however, it can be converted from one form to another. [9-12]
Engineering Connection
Materials List
- Water gun with a pumping mechanism (for example, Super Soaker Water Blasters; available at stores such as Target, Kmart, WalMart or online; ~$10 each); ask students to bring in their own, if possible
- Moveable desk or table, on which to mount the water gun
- Duct tape
- Access to a tape measure long enough to measure 35+ feet (11+ meters), the shot distances
- (optional) Chalk or tape, to mark off every 10 meters of the shooting range
- Pen or pencil
- Take Your Best Shot Worksheet, one per person
- Evaluation & Enhancement Worksheet, one per person
- (optional) Computers and Excel spreadsheet software for data manipulation and graphing
Introduction/Motivation
Procedure
Bernoulli Equation: The Bernoulli equation is an important expression that relates pressure, height and velocity of a fluid at one point along its flow. According to the Venturi Effect, a fluid’s pressure decreases as its velocity increases. The Bernoulli equation puts this relationship into mathematical terms and includes a term for fluid height. Think of water moving down a water slide. At the top (where you load), the water is slow moving, pushed only by the water behind it. When the slide drops, the water rushes down quickly, increasing speed as it falls. Thus, the velocity is also affected by gravity through height. When all these terms are related and scaled for density and gravity, we have the Bernoulli equation,





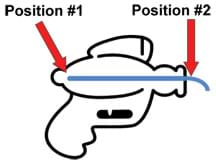
Copyright © 2009 ITL Program, College of Engineering, University of Colorado at Boulder.
Before the Activity
- Gather materials. If possible, ask students to bring their own super soaker water guns from home.
- Make copies of the Take Your Best Shot Worksheet and the Evaluation & Enhancement Worksheet.
- Find an appropriate location to shoot the squirt guns. It is best to secure a relatively open space with access to water and a dry concrete surface (to better see where water lands).
- Set up a table or desk so it is level, and mark off with chalk or tape the distance from the table every meter for ~10 meters.
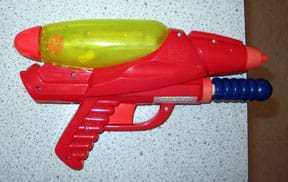
Copyright © 2009 James Prager, ITL Program, College of Engineering, University of Colorado at Boulder.
With the Students
- Conduct a pre-activity assessment discussion with the students, as described in the Assessment section.
- Have students read the background and procedural instructions on the Take Your Best Shot Worksheet, and set up their experiments.
- When ready, run the experiments as described in the worksheet.
- Have the students perform the calculations and graphing on the Take Your Best Shot Worksheet. (Optional: Have them use a digital spreadsheet such as Microsoft Excel for data manipulation and graphing.)
- Have students complete the Evaluation & Enhancement Worksheet.
Attachments
- Take Your Best Shot Worksheet (doc)
- Take Your Best Shot Worksheet (pdf)
- Take Your Best Shot Worksheet Answers (doc)
- Take Your Best Shot Worksheet Answers (pdf)
- Evaluation & Enhancement Worksheet (doc)
- Evaluation & Enhancement Worksheet (pdf)
- Evaluation & Enhancement Worksheet Answers (doc)
- Evaluation & Enhancement Worksheet Answers (pdf)
Safety Issues
- Do not aim squirt guns at eyes.
Troubleshooting Tips
- Depending on your exact water gun design, it is probably best to place the water gun sideways on a flat table to ensure a horizontal shot and easy trigger access.
- One or two pumps might not yield any results depending on the type of water gun used. If this is the case, have students start their data collection at the first number of pumps that yield results and perform trials at up to ten pumps beyond that.
- It is important to leave some air in the water tank to make sure the water gun works properly.
- If holding the trigger until the water stops shooting does not work, the depressurization of the chamber between trials can be achieved by opening the water tank, thereby depressurizing the system.
Assessment
Pre-Activity Assessment
- Most popular water guns today use a hand pump to build up air pressure and shoot water out of a tube, often in order to soak your friends and family while looking totally awesome. How much pressure do you think it takes to have this fun? How would one even go about calculating that? The following activity answers those questions, but first, what do you think?
Activity Embedded Assessment
Post-Activity Assessment
Activity Scaling
- For lower grades, have students calculate and graph the pressure generated by five pumps instead of 10. Also, depending on students’ math ability, provide them with more help in doing the calculations. For instance, provide the equations in order of use and rearrange each one so that the unknowns are on the left sides, requiring minimal algebraic manipulation.
- For higher grades, have students work out the problem solution more independently and require more analysis of the results.
Additional resources
- African-American Inventors. Several eGFI teachers features, including Garrett Morgan, inventor of the traffic signal,
- Bernoulli’s Principle. NASA lessons, hands-on activities, and history of 18th-century scientist Daniel Bernoulli for student in grades 5 to 8. [PDF]
- Bernoulli’s Principle Companion TeachEngineering. org activity for high school students.
- History of the Super Soaker. Brief history and timeline of the popular water gun’s development and its inventor, mechanical engineer Lonnie Johnson, Jr.
- Who Made That Super Soaker? The 2013 New York Times Magazine feature includes a q & a with a University of Texas fluid dynamics Ph.D. student who designs high-power squirt guns.
- Super Soaker Project-Based Math Activity This standards-based high-school lesson from Worcester Polytechnic Institute’s Center for Industrial Mathematics and Statistics lets students apply algebra, gather data, develop and interpret graphs, plot linear equations, and calculate percents. Click HERE for other math projects.
Bernoulli’s principle. Wikipedia, The Free Encyclopedia. Accessed February 21, 2016.
Knight, Randall. Physics for Scientists and Engineers: A Strategic Approach. Second edition. San Francisco, CA: Pearson Addison-Wesley, 2008.
Munson, B. R., Young, D.F., Okiishi, T.H., Fundamentals of Fluid Mechanics. Fifth edition. New York, NY: John Wiley & Sons, Inc., 2006.
Contributed by by James Prager, Karen King, Denise W. Carlson © 2009 by Regents of the University of Colorado.
Supporting Program: Integrated Teaching and Learning Program, College of Engineering, University of Colorado Boulder
Filed under: Class Activities, Grades 9-12 | Comments Off on A Shot Under Pressure